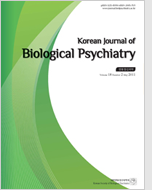
- Past Issues
- e-Submission
-
2021 Impact Factor 1.766
5-Year Impact Factor 1.674
Editorial Office
- +82-01-9989-7744
- kbiolpsychiatry@gmail.com
- https://www.biolpsychiatry.or.kr/
2021 Impact Factor 1.766
5-Year Impact Factor 1.674
Korean Journal of Biological Psychiatry 2015;22(1):20-7. Published online: Jan, 1, 2015
Objectives :
It is increasingly thought that the human cerebellum plays an important role in emotion and cognition. Although recent evidence suggests that the cerebellum may also be implicated in fear learning, only a limited number of studies have investigated the cerebellar abnormalities in panic disorder. The aim of this study was to evaluate the cerebellar gray matter deficits and their clinical correlations among patients with panic disorder.
Methods : Using a voxel-based morphometry approach with a high-resolution spatially unbiased infratentorial template, regional cerebellar gray matter density was compared between 23 patients with panic disorder and 33 healthy individuals.
Results : The gray matter density in the right posterior-superior (lobule Crus I) and left posterior-inferior (lobules Crus II, VIIb, VIIIa) cerebellum was significantly reduced in the panic disorder group compared to healthy individuals (p < 0.05, false discovery rate corrected, extent threshold = 100 voxels). Additionally, the gray matter reduction in the left posterior-inferior cerebellum (lobule VIIIa) was significantly associated with greater panic symptom severity (r = -0.55, p = 0.007).
Conclusions : Our findings suggest that the gray matter deficits in the posterior cerebellum may be involved in the pathogenesis of panic disorder. Further studies are needed to provide a comprehensive understanding of the cerebro-cerebellar network in panic disorder.
Keywords Panic disorder;Cerebellum;Voxel-based morphometry;Gray matter.
Address for correspondence: Soo Mee Lim, MD, Department of Radiology, Ewha Womans University Mokdong Hospital, 1071 Anyangcheon-ro, Yangcheon-gu, Seoul 158-710, Korea
Tel: +82-2-2650-6022, Fax: +82-2-2650-2838, E-mail: soomee@ewha.ac.kr
*These authors contributed equally to this work.
ㅔPanic disorder is characterized by composite features of recurrent panic attacks, anticipatory anxiety, cognitive distortion, and avoidance behaviors.1) Several neuroimaging studies of the human brain have demonstrated that significant functional and/or structural alterations in cerebral regions including the temporal lobe (the amygdala, hippocampus, insula, and parahippocampal gyrus), prefrontal cortex, and cingulate cortex, are related to panic disorder.2)3)4)5)6) These cerebral regions are known to mediate fear response, which may play a major role in the pathogenesis of panic disorder.7)
ㅔAlong with the advance in neuroimaging techniques, an increasing number of studies have revealed that the cerebellum modulates emotion and cognition as well as motor coordination.8)9)10)11)12)13) Furthermore, the extensive cerebro-cerebellar connections may be a part of the neural circuits involved in emotional and cognitive processing during associative learning.14)15)16)17) Recent studies have also reported the cerebellar abnormalities in several neuropsychiatric disorders, including schizophrenia, mood disorder, and developmental disorder.18)19)20)21)
ㅔDespite mounting evidence of cerebellar involvement in emotion and cognition, there are no reports focusing solely on the cerebellum in panic disorder. Up till now, the structural and functional deficits in the cerebellum have been reported merely as unexpected findings in panic disorder.22)23)24)25)26) It is notable, however, that higher glucose metabolism in the cerebellum along with fear network-related cerebral regions was found in patients with panic disorder.22) These alterations of glucose metabolism in the brain including the cerebellum were normalized after successful cognitive-behavioral therapy.23)
ㅔThere is increasing evidence that the cerebellum takes part in the fear circuitry by playing a role in associative learning.27)28) Several studies have shown impaired fear conditioning in patients with cerebellar damage.29)30) Moreover, recent functional neuroimaging studies have reported increased activation in the posterior cerebellum during anticipation of an aversive stimulus.31)32)33)
ㅔTaken together, we hypothesized that the structural deficits in the cerebellum would contribute to pathogenesis of panic disorder. We aimed to investigate the differences in cerebellar gray matter density between patients with panic disorder and healthy individuals, using a voxel-based morphometry (VBM)34) method with a high-resolution atlas template of the human cerebellum.35)36) We were also interested in assessing the association of the cerebellar gray matter deficits with current panic symptom severity.
Methods
Participants
ㅔTwenty-three patients with panic disorder were recruited from an outpatient clinic in Seoul, South Korea (Department of Neuropsychiatry, Seoul National University Hospital). Panic disorder was diagnosed using the Structured Clinical Interview for DSM-IV (SCID-IV).37) Thirty-three healthy individuals were recruited through local newspaper advertisement. Subjects for both groups who met any of the following criteria were excluded: 1) diagnosed with any other Axis I disorder by SCID-IV interview, 2) antisocial or borderline personality disorder screened by Personality Disorder Questionnaire-IV,38) 3) current or past history of major medical or neurological illness, and 4) having any contraindication to magnetic resonance imaging (MRI) scanning. After providing information about the study, written informed consent was obtained from all participants. Approval for this study was granted by the ethics committee of the Seoul National University Hospital (H-0805-080-245).
ㅔBasic demographic data were collected for all participants, including age, sex, and handedness. The Panic Disorder Severity Scale (PDSS)39)40) and the Zung Self-Rating Anxiety Scale (Z-SAS)41) were used to assess symptom severity in the panic disorder group. Comorbid depressive symptoms were assessed with the Hamilton Depression Rating Scale.42) Neuropsychological tests including the Digit Symbol Substitution Test, the Digit Span Forward and Backward Test, the Spatial Span Forward and Backward Test, the Trail Making Test-A (TMT-A) and the Trail Making Test-B (TMT-B) were performed in all participants.
Magnetic resonance image acquisition
ㅔA three-dimensional spoiled gradient echo pulse sequence was used on a 3.0-T GE VHi scanner (GE Medical System, Milwaukee, WI, USA) and 248 sagittal T1 images were acquired (repetition time = 5.7 ms, echo time = 1.4 ms, inversion time = 400 ms, slice thickness = 0.7 mm, matrix size = 256 × 256, field of view = 22 cm, flip angle = 20°, number of excitation = 1).
Image preprocessing and voxel-based morphometry
ㅔAll of the image processing was conducted using Statistical Parametric Mapping (SPM 5 : Wellcome Department of Cognitive Neurology, University College London, UK) run on MATLAB (Math Works, Natick, MA, USA). Before the processing, T1 weighted images were reoriented and the origin was set to the anterior commissure by MRIcro (http://www.mricro.com) and resliced to a 1mm isovoxel image by Analyze 5.0. Because the Spatially Unbiased Infratentorial (SUIT) template can provide a more accurate inter-subjects alignment of the cerebellum, we used the SUIT toolbox version 2.4 (available at http://www.icn.ucl.ac.kr/motorcontrol/imaging/suit.htm)35) in the following processing. Using the isolate function within the SUIT toolbox, the infratentorial structures including the cerebellum and brainstem were cropped. Then, the cropped individual images were normalized into the SUIT template.34) The deformation map produced in the process was used to reslice and segment the gray matter of the cerebellum.35) The segmented images were modulated to preserve the actual proportion of gray matter within a given voxel.35) Each step was carefully reviewed by an experienced researcher (J.Y.J) before progressing into the next step. The final modulated images were smoothed using 4-mm full width at half-maximum isotropic Gaussian kernel.
Statistical analysis
ㅔGroup differences in demographic and clinical characteristics were assessed using the independent t-test for continuous variables and the chi-square test or the
Fisher's exact test for categorical variables. The smoothed images were statistically analyzed using the general linear model and random Gaussian field theory.43) To compare the cerebellar gray matter density between the two groups, we conducted an analysis of covariance using SPM5. We performed global normalisation with proportional scaling, which removes global gray matter differences for each participant. Age and sex were treated as confounding covariates. Statistical analysis was carried out using a general linear model embedded in SPM5. The limit for statistical significance was set at p
< 0.05 after false discovery rate correction and an extent threshold of 100 contiguous voxels.
ㅔTo investigate the relationships between regional cerebellar gray matter deficits and panic disorder, we calculated the
Pearson's correlation coefficients between the mean gray matter density and the clinical characteristics including panic symptom severity and neuropsychological deficits. The mean gray matter density was derived from the first eigenvariates of the clusters showing significant group differences in a VBM analysis. Statistical analysis was performed by Stata 12.1 (StataCorp, College Station, TX, USA). The significance level was defined at an alpha level of 0.05. To account for multiple testing of each cluster, the Bonferroni correction (alpha = 1-0.951/n ; n = number of significant clusters) was applied.
Results
Characteristics of participants
ㅔThe demographic and clinical characteristics of the study participants and between-group comparison results are summarized in Table 1. There were no statistically significant differences in age, sex composition, and handedness between the two groups. The mean score on the PDSS and the Z-SAS in patients with panic disorder was 9.2 ± 6.2 and 42.8 ± 9.7, respectively.
Comparisons of gray matter density between the panic disorder group and healthy individuals
ㅔAfter adjusting for the confounding variables including age and sex, we found five clusters of significantly reduced gray matter density in the panic disorder group as compared to healthy individuals (Table 2, Fig. 1). The largest cluster was located in the right posterior-superior cerebellum [lobule Crus I, peak voxel (20, -81, -26), cluster size 1821 voxels, corrected p
< 0.001]. The other four clusters were found in the left posterior-inferior cerebellum [lobule Crus II, peak voxel (-44, -64, -54), cluster size 144 voxels, corrected p = 0.006 ; lobule Crus II, peak voxel (-37, -78, -42), cluster size 374 voxels, corrected p = 0.015 ; lobule VIIb, peak voxel (-27, -68, -58), cluster size 113 voxels, p = 0.022 ; lobule VIIIa, peak voxel (-25, -58, -61), cluster size 163 voxels, p = 0.023]. There were no regions of increased gray matter density in the panic disorder group.
Relationship between cerebellar gray matter deficits and clinical characteristics
ㅔWithin-group analysis of patients with panic disorder showed a significant correlation between the PDSS scores and gray matter density reduction in the lobule VIIIa (r = -0.55, p = 0.007)(Fig. 2). The PDSS scores were not significantly correlated with the gray matter density in any other clusters (cluster in the lobule Crus I, r = -0.08, p = 0.729 ; clusters in the lobule Crus II, r = -0.46, p = 0.026 and r = -0.32, p = 0.132 ; cluster in the lobule VIIb, r = -0.41, p = 0.051).
ㅔCorrelations between the neuropsychological tests and the regional gray matter density in the panic disorder group are shown in Table 3. The total time taken to complete the TMT-A was negatively correlated with the gray matter density within both the clusters in the left Crus II (r = -0.65, p
< 0.001 ; r = -0.57, p = 0.004). The Digit Symbol Substitution Test scores and the Digit Span Forward Test scores were positively correlated with the gray matter density in both the left Crus II (r = 0.57, p = 0.005 ; r = 0.63, p = 0.001, respectively) and the left lobule VIIb (r = 0.61, p = 0.002 ; r = 0.57, p = 0.005, respectively). There was also a positive correlation between the Spatial Span Forward scores and the gray matter density in the left Crus II (r = 0.57, p = 0.005). No correlations were found between the neuropsychological tests and the cerebellar gray matter density in healthy individuals.
Discussion
ㅔOur study investigated the cerebellar gray matter deficits and their clinical correlations among patients with panic disorder. As hypothesized, the cerebellar gray matter density in the posterior-superior and posterior-inferior lobes was markedly reduced in the panic disorder group compared with healthy individuals. Additionally, the cerebellar gray matter deficits in the posterior-inferior lobe were significantly associated with greater panic symptoms severity and lower neuropsychological function among patients with panic disorder. The relationship between cerebellar regions and panic disorder has not been previously established. To the best of our knowledge, this is the first study to focus on the structural abnormalities in the cerebellum in panic disorder by employing the SUIT template for an accurate alignment of the infra-tentorial structures.35)
ㅔThe cerebellar cortex can be divided into anterior (lobules I-V), posterior-superior (lobule VI/Crus I) and posterior-inferior (lobule VIIa-X) lobes by the primary and horizontal fissures.44) In our study, the cerebellar gray matter deficits were found in the posterior-superior and the posterior-inferior lobes but not in the anterior lobe. These results may be congruent with the findings from previous studies of functional topography in the human cerebellum.11)13) The anterior lobe is known to be involved in motor coordination, while the posterior lobe, which is anatomically and functionally connected to the prefrontal and parietal cortices, is believed to be involved in regulating emotion and cognition.11)13)
ㅔFrom medial to lateral, the cerebellum can also be divided into the vermis and the hemispheres.44) Among these subregions, the posterior vermal regions have been extensively investigated with respect to fear response. Several animal studies have reported that the posterior vermal regions are involved in the fear conditioning.45)46) Furthermore, increased activation in the vermal regions was found during cholecystokinin tetrapeptide- or lactate-induced panic attacks among healthy volunteers.25)47)48)49) Recent studies, however, suggest that the posterior cerebellar hemispheres are also likely to play a potential role in the fear conditioning process.11)28) Moers-Hornikx et al.50) reported that panic-like behaviors in rats were accompanied by deactivation of the dentate nucleus within the posterior cerebellar hemispheres, as well as the fastigial nucleus in the vermis. A meta-analysis of functional neuroimaging studies revealed the different roles of the posterior vermis, which is involved in emotional processing and the posterior hemispheres, participating in cognitive processing in the human cerebellum. Another recent review of the human cerebellar lesion studies showed that the vermal regions are important in autonomic and somatic responses to fear, whereas the posterior cerebellar hemispheres contribute to emotional and cognitive associative learning of fear,28) which is one of the main components of panic disorder.51)52)
ㅔOur study showed the gray matter deficits only in the posterior hemispheres, but not in the posterior vermis. Considering the previous studies that reported increased activation of the vermis during a panic attack but not during anticipatory anxiety,25)47)48)49) a possible explanation is that the vermal deficits may be related to the panic attack itself. Nevertheless, with a small sample size, caution must be exercised and further studies are needed to verify the different roles of the vermis and the cerebellar hemispheres in panic disorder.
ㅔIn the current study, a significant correlation of cerebellar deficits in the lobule VIIIa with symptom severity was observed in patients with panic disorder. Interestingly, this finding is concordant with that in a previous VBM study showing gray matter deficits in the lobule VIIIa as one of the putative state markers in major depressive disorder with panic disorder.26) This study reported duloxetine-induced changes in gray matter density in several brain areas, including the cerebellar lobule VIIIa among drug-naïve major depressive disorder with panic disorder patients.26)
ㅔThe lobule VIIIa may be involved in modulation and storage of information.53) It is notable that the cerebello-parietal loop between the lobule VIIIa and the inferior parietal lobe is involved in encoding and storage of relevant information during working memory task.53)54) Furthermore, functional MRI activation in the lobule VIIIa was linearly increased with higher memory load and practice-related proficiency.54) Considering that emotional associative learning such as fear conditioning may impose higher cognitive load55) structural deficits in the lobule VIIIa could induce failure of adaptive conditioned response and contribute to the development of panic symptoms.
ㅔWe also found significant correlations between the gray matter deficits in the posterior-inferior lobe and cognitive performances including memory and information processing, which is mostly in accordance with the results of previous studies of functional topography in the cerebellum.11) Ravizza et al.56) also suggested that the extent of damage to the posterior-inferior cerebellum was associated with the storage of working memory. Furthermore, as mentioned above, the up-to-date research has confirmed that the posterior-inferior hemispheres in the cerebellum are crucial for adaptive learning of emotion and cognition.28) These findings enhance our understanding of the role of the posterior-inferior cerebellum in patients with panic disorder. Taken together, it could be conceivably hypothesized that the cerebellar deficits in the posterior-inferior lobe may be associated with impairment of emotional and cognitive associative learning, and consequent failure to select relevant information and response with adequate behavior in panic disorder.
ㅔHowever, more research on this hypothesis needs to be undertaken before these assertions can be more clearly understood, as some of our findings in this study do not support those of the previous research. Several neuroimaging studies suggest that the posterior-superior cerebellar lobe including the lobule VI and the Crus I also play a role in eye-blink conditioning and associative learning. In spite of the largest cluster of gray matter deficits in the posterior-superior lobe, no significant associations were found with current panic symptoms in this study.
ㅔAmong several studies using a whole-brain VBM analysis in panic disorder, only a few studies have reported structural deficits in the cerebellum.22)24)26) The negative findings of previous structural neuroimaging studies could, in part, be due to the insufficiency of the whole-brain template to provide accurate spatial normalization of the cerebellum.35) To address this issue, the current study used the SUIT approach that provides high sensitivity and accuracy in the analysis of the cerebellum.35)57) However, since the focus of the current study is on the structural abnormalities in the cerebellum, our findings have limitations in providing a comprehensive view of the cerebellar-cerebral network within the neural mechanisms of panic disorder. In addition, the current or previous use of psychotropic medication may bring potential confounding effects on cerebellar gray matter deficits.26)58)
ㅔOur previous report using the whole-brain VBM analysis in this sample showed decreased gray matter volumes in the basal ganglia but not in the cerebellum.59) Interconnections between the cerebellum and the basal ganglia have long been implicated in the manifestation of motor disorders, through their involvement in associative learning.60) Interestingly, recent evidences suggest that the cerebellar interactions with the basal ganglia also contribute to regulation of emotion and cognition.60) Hence, further work is needed to explore how interactions between the cerebellum and the cerebrum contribute to symptoms of panic disorder.
American Psychiatric Association. Diagnostic and statistical manual of mental disorders: DSM-IV-TR. 4th ed., text revision. Washington, DC: American Psychiatric Association;2000.
Massana G, Serra-Grabulosa JM, Salgado-Pineda P, Gastó C, Junqué C, Massana J, et al. Amygdalar atrophy in panic disorder patients detected by volumetric magnetic resonance imaging. Neuroimage 2003;19:80-90.
Pillay SS, Gruber SA, Rogowska J, Simpson N, Yurgelun-Todd DA. fMRI of fearful facial affect recognition in panic disorder: the cingulate gyrus-amygdala connection. J Affect Disord 2006;94:173-181.
Han DH, Renshaw PF, Dager SR, Chung A, Hwang J, Daniels MA, et al. Altered cingulate white matter connectivity in panic disorder patients. J Psychiatr Res 2008;42:399-407.
Chechko N, Wehrle R, Erhardt A, Holsboer F, Czisch M, Sämann PG. Unstable prefrontal response to emotional conflict and activation of lower limbic structures and brainstem in remitted panic disorder. PLoS One 2009;4:e5537.
Yoon S, Jun CS, Jeong HS, Lee S, Lim SM, Ma J, et al. Altered cortical gyrification patterns in panic disorder: deficits and potential compensation. J Psychiatr Res 2013;47:1446-1454.
Gorman JM, Kent JM, Sullivan GM, Coplan JD. Neuroanatomical hypothesis of panic disorder, revised. Am J Psychiatry 2000;157:493-505.
Exner C, Weniger G, Irle E. Cerebellar lesions in the PICA but not SCA territory impair cognition. Neurology 2004;63:2132-2135.
Schmahmann JD, Weilburg JB, Sherman JC. The neuropsychiatry of the cerebellum - insights from the clinic. Cerebellum 2007;6:254-267.
Tavano A, Grasso R, Gagliardi C, Triulzi F, Bresolin N, Fabbro F, et al. Disorders of cognitive and affective development in cerebellar malformations. Brain 2007;130(Pt 10):2646-2660.
Stoodley CJ, Schmahmann JD. Functional topography in the human cerebellum: a meta-analysis of neuroimaging studies. Neuroimage 2009;44:489-501.
Stoodley CJ, Valera EM, Schmahmann JD. An fMRI study of intra-individual functional topography in the human cerebellum. Behav Neurol 2010;23:65-79.
Stoodley CJ, Schmahmann JD. Evidence for topographic organization in the cerebellum of motor control versus cognitive and affective processing. Cortex 2010;46:831-844.
Ramnani N. The primate cortico-cerebellar system: anatomy and function. Nat Rev Neurosci 2006;7:511-522.
Ramnani N. Frontal lobe and posterior parietal contributions to the cortico-cerebellar system. Cerebellum 2012;11:366-383.
Ito M. Control of mental activities by internal models in the cerebellum. Nat Rev Neurosci 2008;9:304-313.
Moulton EA, Elman I, Pendse G, Schmahmann J, Becerra L, Borsook D. Aversion-related circuitry in the cerebellum: responses to noxious heat and unpleasant images. J Neurosci 2011;31:3795-3804.
Nopoulos PC, Ceilley JW, Gailis EA, Andreasen NC. An MRI study of cerebellar vermis morphology in patients with schizophrenia: evidence in support of the cognitive dysmetria concept. Biol Psychiatry 1999;46:703-711.
Okugawa G, Sedvall G, Nordström M, Andreasen N, Pierson R, Magnotta V, et al. Selective reduction of the posterior superior vermis in men with chronic schizophrenia. Schizophr Res 2002;55:61-67.
De Bellis MD, Kuchibhatla M. Cerebellar volumes in pediatric maltreatment-related posttraumatic stress disorder. Biol Psychiatry 2006;60:697-703.
Monkul ES, Hatch JP, Sassi RB, Axelson D, Brambilla P, Nicoletti MA, et al. MRI study of the cerebellum in young bipolar patients. Prog Neuropsychopharmacol Biol Psychiatry 2008;32:613-619.
Sakai Y, Kumano H, Nishikawa M, Sakano Y, Kaiya H, Imabayashi E, et al. Cerebral glucose metabolism associated with a fear network in panic disorder. Neuroreport 2005;16:927-931.
Sakai Y, Kumano H, Nishikawa M, Sakano Y, Kaiya H, Imabayashi E, et al. Changes in cerebral glucose utilization in patients with panic disorder treated with cognitive-behavioral therapy. Neuroimage 2006;33:218-226.
Asami T, Yamasue H, Hayano F, Nakamura M, Uehara K, Otsuka T, et al. Sexually dimorphic gray matter volume reduction in patients with panic disorder. Psychiatry Res 2009;173:128-134.
Eser D, Leicht G, Lutz J, Wenninger S, Kirsch V, Schüle C, et al. Functional neuroanatomy of CCK-4-induced panic attacks in healthy volunteers. Hum Brain Mapp 2009;30:511-522.
Lai CH, Hsu YY. A subtle grey-matter increase in first-episode, drug-naive major depressive disorder with panic disorder after 6 weeks' duloxetine therapy. Int J Neuropsychopharmacol 2011;14:225-235.
Kim JJ, Jung MW. Neural circuits and mechanisms involved in Pavlovian fear conditioning: a critical review. Neurosci Biobehav Rev 2006;30:188-202.
Timmann D, Drepper J, Frings M, Maschke M, Richter S, Gerwig M, et al. The human cerebellum contributes to motor, emotional and cognitive associative learning. A review. Cortex 2010;46:845-857.
Maschke M, Drepper J, Kindsvater K, Kolb FP, Diener HC, Timmann D. Involvement of the human medial cerebellum in long-term habituation of the acoustic startle response. Exp Brain Res 2000;133:359-367.
Maschke M, Schugens M, Kindsvater K, Drepper J, Kolb FP, Diener HC, et al. Fear conditioned changes of heart rate in patients with medial cerebellar lesions. J Neurol Neurosurg Psychiatry 2002;72:116-118.
Ploghaus A, Tracey I, Clare S, Gati JS, Rawlins JN, Matthews PM. Learning about pain: the neural substrate of the prediction error for aversive events. Proc Natl Acad Sci U S A 2000;97:9281-9286.
Ploghaus A, Tracey I, Gati JS, Clare S, Menon RS, Matthews PM, et al. Dissociating pain from its anticipation in the human brain. Science 1999;284:1979-1981.
Damasio AR, Grabowski TJ, Bechara A, Damasio H, Ponto LL, Parvizi J, et al. Subcortical and cortical brain activity during the feeling of self-generated emotions. Nat Neurosci 2000;3:1049-1056.
Ashburner J, Friston KJ. Voxel-based morphometry--the methods. Neuroimage 2000;11(6 Pt 1):805-821.
Diedrichsen J. A spatially unbiased atlas template of the human cerebellum. Neuroimage 2006;33:127-138.
Diedrichsen J, Balsters JH, Flavell J, Cussans E, Ramnani N. A probabilistic MR atlas of the human cerebellum. Neuroimage 2009;46:39-46.
First MB, Spitzer RL, Gibbon M, Williams JBW. Structured clinical interview for DSM-IV axis I disorders (SCID-I). Washington, DC: American Psychiatric Press;1997.
Hyler SE. PDQ-4+ Personality Questionnaire. New York: New York State Psychiatric Institute;1994.
Shear MK, Rucci P, Williams J, Frank E, Grochocinski V, Vander Bilt J, et al. Reliability and validity of the Panic Disorder Severity Scale: replication and extension. J Psychiatr Res 2001;35:293-296.
Lim YJ, Yu BH, Kim JH. Korean panic disorder severity scale: construct validity by confirmatory factor analysis. Depress Anxiety 2007;24:95-102.
Zung WW. A rating instrument for anxiety disorders. Psychosomatics 1971;12:371-379.
Hamilton M. A rating scale for depression. J Neurol Neurosurg Psychiatry 1960;23:56-62.
Friston KJ, Worsley KJ, Frackowiak RS, Mazziotta JC, Evans AC. Assessing the significance of focal activations using their spatial extent. Hum Brain Mapp 1994;1:210-220.
Larsell O, Jansen J. The Comparative Anatomy and Histology of the Cerebellum: from monotremes through apes. Minneapolis: University of Minnesota Press;1967.
Sacchetti B, Scelfo B, Strata P. The cerebellum: synaptic changes and fear conditioning. Neuroscientist 2005;11:217-227.
Sacchetti B, Baldi E, Lorenzini CA, Bucherelli C. Cerebellar role in fear-conditioning consolidation. Proc Natl Acad Sci U S A 2002;99:8406-8411.
Javanmard M, Shlik J, Kennedy SH, Vaccarino FJ, Houle S, Bradwejn J. Neuroanatomic correlates of CCK-4-induced panic attacks in healthy humans: a comparison of two time points. Biol Psychiatry 1999;45:872-882.
Schunck T, Erb G, Mathis A, Gilles C, Namer IJ, Hode Y, et al. Functional magnetic resonance imaging characterization of CCK-4-induced panic attack and subsequent anticipatory anxiety. Neuroimage 2006;31:1197-1208.
Reiman EM. The application of positron emission tomography to the study of normal and pathologic emotions. J Clin Psychiatry 1997;58 Suppl 16:4-12.
Moers-Hornikx VM, Vles JS, Lim LW, Ayyildiz M, Kaplan S, Gavilanes AW, et al. Periaqueductal grey stimulation induced panic-like behaviour is accompanied by deactivation of the deep cerebellar nuclei. Cerebellum 2011;10:61-69.
Grillon C. Associative learning deficits increase symptoms of anxiety in humans. Biol Psychiatry 2002;51:851-858.
Grillon C, Lissek S, McDowell D, Levenson J, Pine DS. Reduction of trace but not delay eyeblink conditioning in panic disorder. Am J Psychiatry 2007;164:283-289.
Chen SH, Desmond JE. Cerebrocerebellar networks during articulatory rehearsal and verbal working memory tasks. Neuroimage 2005;24:332-338.
Kirschen MP, Chen SH, Schraedley-Desmond P, Desmond JE. Load- and practice-dependent increases in cerebro-cerebellar activation in verbal working memory: an fMRI study. Neuroimage 2005;24:462-472.
Carter RM, Hofstotter C, Tsuchiya N, Koch C. Working memory and fear conditioning. Proc Natl Acad Sci U S A 2003;100:1399-1404.
Ravizza SM, McCormick CA, Schlerf JE, Justus T, Ivry RB, Fiez JA. Cerebellar damage produces selective deficits in verbal working memory. Brain 2006;129(Pt 2):306-320.
Morrell MJ, Jackson ML, Twigg GL, Ghiassi R, McRobbie DW, Quest RA, et al. Changes in brain morphology in patients with obstructive sleep apnoea. Thorax 2010;65:908-914.
Lai CH. Duloxetine related effects of brain structure on a patient of major depressive disorder with panic disorder. Prog Neuropsychopharmacol Biol Psychiatry 2010;34:240-241.
Yoo HK, Kim MJ, Kim SJ, Sung YH, Sim ME, Lee YS, et al. Putaminal gray matter volume decrease in panic disorder: an optimized voxel-based morphometry study. Eur J Neurosci 2005;22:2089-2094.
Bostan AC, Dum RP, Strick PL. Cerebellar networks with the cerebral cortex and basal ganglia. Trends Cogn Sci 2013;17:241-254.