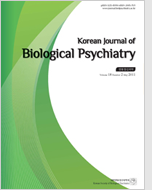
- Past Issues
- e-Submission
-
2021 Impact Factor 1.766
5-Year Impact Factor 1.674
Editorial Office
- +82-01-9989-7744
- kbiolpsychiatry@gmail.com
- https://www.biolpsychiatry.or.kr/
2021 Impact Factor 1.766
5-Year Impact Factor 1.674
Korean Journal of Biological Psychiatry 2023;30(1):1-6. Published online: Apr, 1, 2023
DOI : https://doi.org/10.22857/kjbp.2023.30.1.001
Many psychiatric disorders are associated with brain functional dysfunctions and neuronal degeneration. According to the research so far, enhanced brain plasticity reduces neurodegeneration and recovers neuronal damage. Brain-derived neurotrophic factor (BDNF) is one of the most extensively studied neurotrophins in the mammalian brain that plays major roles in neuronal survival, development, growth, and maintenance of neurons in brain circuits related to emotion and cognitive function. Also, BDNF plays an important role in brain plasticity, influencing dendritic spines in the hippocampus neurogenesis. Changes in neurogenesis and dendritic density can improve psychiatric symptoms and cognitive functions. BDNF has potent effects on brain plasticity through biochemical mechanisms, cellular signal pathways, and epigenetic changes. There are pharmacological and non-pharmacological interventions to increase the expression of BDNF and enhance brain plasticity. Non-pharmacological interventions such as physical exercise, nutritional change, environmental enrichment, and neuromodulation have biological mechanisms that increase the expression of BDNF and brain plasticity. Non-pharmacological interventions are cost-effective and safe ways to improve psychiatric symptoms.
Keywords Brain plasticity; BDNF; Complementary Therapies.
Address for correspondence: Hyun Kook Lim, MD, PhD Department of Psychiatry, Yeouido St. Mary’s Hospital, College of Medicine, The Catholic University of Korea, 10, 63-ro, Yeongdeungpo-gu, Seoul 07345, Korea
Tel: +82-2-3779-1048, Fax:+82-2-780-6577, E-mail: drblues@catholic.ac.kr
서 론
조현병, 우울증, 양극성 장애, 알츠하이머병 등 정신과 질환 은 신경세포의 기능장애나 변성, 사멸로 인해 발생한다.1) 고령사회로 진입하고 있는 상황에서 노화로 인한 신경변성장애 환자의 증가도 매우 중요한 건강관리의 영역이다. 신경세포의 기능장애, 변성, 사멸 등을 예방하고 이를 회복할 수 있는 뇌신경가소성이 대두되고 있다.2) 뇌 신경가소성에 관여하는 많은 인자들 중 뇌 유래신경영양인자(brain-derived neuro-trophic factor, BDNF)가 가장 많이 연구되었으며 BDNF가 신경세포의 발달, 성장, 생존, 유지 등에 긍정적인 영향을 주고 뇌 신경가소성을 향상시키며 이를 통해 뇌 기능적 향상을 유도하는 작용 기전에 대해서는 많이 밝혀졌다. 3)
리튬(lithium), 선택적 세로 토닌 재흡수 억제제(selective serotonin reuptake inhibitor, SSRI), 케타민(ketamine) 같은 정신과 약물의 사 용으로 BDNF를 증가시킬 수 있다.4-6) 실제 임상환경에서 약물적 치료 이외에 생활 습관 교정, 환자 교육과 같은 비약 물적 개입을 통해 치료 효과를 더 높일 수 있다.7)8) 또한 비약 물적 개입은 부작용의 가능성이 낮으며 매우 효율적으로 적용될 수 있다. 이에 본 종설에서 BDNF가 뇌 신경가소성에 작용하는 생물학적 기전을 확인하고 이를 증가 시킬 수 있는 비약 물적 접근에 대해 고찰해보고자 한다.
뇌신경 세포는 출생에서 사망에 이르기까지 시기에 따라 생장, 분화, 사멸이 일어난다. 영유아기에는 생장과 분화, 시냅스의 형성이 주로 일어나고 성인기에 이르러서는 조금씩 사멸의 과정으로 진행된다. 따라서 노화의 과정에서 뇌세포의 사멸과 시냅스의 기능저하들로 인해 인지기능 저하 및 운동기능의 저하가 발생한다. 하지만 이러한 일련의 과정에서 뇌 신경가소성의 활성화를 통해 신경세포의 사멸을 늦추거나 보상할 수 있다는 사실은 많이 알려져 있다.2) 뇌 신경가소성은 우선 시냅스의 기능을 강화 시킴으로써 학습 및 기억 생성 과정,2) 그리고 손상되거나 사멸한 뉴런을 보상 하기 위해 새로운 뉴런이 형성되는 것9) 두 가지 형태로 발현된다.
성인의 뇌 신경가소성을 향상시키는 여러 물질, 신경영양인자(neurotrophin)들 중에 BDNF가 핵심적인 역할을 하고 있다.10) 이 신경영양인자 는 후성유전학적(epigenetic) 조절을 통해 뉴런의 증식과 분화를 일으키고11) 시냅스의 신경전달을 증가시키며 뇌의 기능적 향상을 일으킨다.12) BDNF 농도의 조절 이상으로 인해 신경세포발생의 문제가 발생하여 치매, 알츠하이머병, 파킨슨병, 조현병, 헌팅턴병, 자폐증, 우울증과 같은 신경과 및 정신건강의학과 질환의 발생 위험이 높아진다는 연구가 있다.13)
BDNF 유전자는 대뇌 해마(hippocampus)의 미세아교세포(microglia), 별아교세포(astrocyte)에서 주로 발현된다. BDNF 유전자의 발현은 세 가지 기전으로 활성화된다. 우선 Nmethyl-D-aspa rtate (NMDA) 수용체의 활성화로 세포내 칼슘이온의 유입으 로 Ca2+/calmoduline dependent protein kinase II (CaMKII)의 활성으로 DNA와 결합하고 있는 히스톤(histone) 단백질의 아세틸화(acetylation)를 유도하여 발현을 증가시킨다. 그리고 BDNF 자체로 Tyrosine receptor kinase B (TrkB) 수 용체에 작용하여 mitogen-activated protein kinase (MAPK) pathway를 통해 cAMP-response element-binding protein (CREB)의 활성화로 BDNF 프로모터의 결합을 증가시켜 유전자 발현을 활성화한다. 마지막으로 에너지 대사로 AMP/ATP ratio가 증가하여 NAD+/NADH ratio가 증가하게 되고 이러한 변화로 sirtuin (SIRTI)을 활성화시켜 BDNF 유전자 발현을 증가시킨다.3)
유전자에서 번역(translation)된 초기 형태의 단백질을 preproBDNF라 하며 이 단백질은 번역 후 변형(post-translational modification)의 과정을 통해 순서대로 proBDN F, mature BDNF로 변형된다. 각각의 proBDNF와 mature BDNF는 작용 기전이 상이하다. proBDNF는 p75NTR에 결합하여 세포 내 신호전달체계를 통해 세포골격 리모델링(cytoskeletal remodeling), 신경세포의 사멸 혹은 생존, 시냅스의 형성을 조절한다.14) Mature BDNF는 TrkB 수 용체와 결합하여 시냅스의 형성, 뉴런의 생존, 신경돌기의 성장을 증가시킨다.15)16) 각각의 작용기전이 다르기에 뇌의 발생과정에 따라 proBDNF와 mature BDNF의 비율이 달라진다. 이를 조절하는 효소로 matrix metalloprotein 2와 9 (MMP-2와 MMP-9)가 작용하고 영유아기에는 proBDNF의 비율이 상대적으로 높아지며 이를 통해 뉴런의 발달, 시냅스의 복잡화, 불필요한 뉴런의 사멸을 일으키고 성인기에는 mature BDNF의 비율이 높아지며 뉴런의 생존, 뇌 신경가소성, 신경돌기의 성장을 증가시킨다.17)
다양한 BDNF 유전자의 다형성(polymorphism)이 발견되었으며 가장 많은 연구가 진행된 것은 66번 아미노산 valine의 methionine 치환이다. Valine 유전자형이 야생형이고, Va l-66Met single nucleotide polymorphism (Val66Met SNP)가 있는 경우 생성된 BDNF의 구조적 변화로 인해 TrkB, NMDA 수용체를 통한 신호전달체계의 감소를 일으켜 BDNF 자체의 생성이 줄어들고 뇌 신경가소성의 기능을 저해한다. 한 쌍의 대립유전자에 MET 유전자형의 수가 많을수록 BDNF의 기능 및 생성이 줄어들게 되고 이는 뇌 신경가소성 및 뇌신경 발달에 이상이 발생할 가능성을 높인다. 또한, 각종 정신 건강의학과질환(우울증, 조현병, 강박장애), 신경변성질환(알츠하이머병, 파킨슨병, 헌팅턴병)에 취약해진다.18)19) 최근 이 SNP의 유무에 따른 항우울제 약물 반응의 차이, 경두개 자기자극술(transcranial magnetic stimulation, TMS), 경두개 직류 자극(transcranial direct current stimulation, tDCS)과 같은 생물학적 치료의 효과 및 부작용의 차이에 대한 연구가 활발히 진행되고 있다.20)21)
신체활동이 뇌 신경가소성에 영향을 주고 많은 정신건강의학과 질환의 예방 및 치료에 도움이 된다는 사실은 많이 알려진 사실이다. 50세 전후 중년의 대상자를 선발하여 21년간 진행된 전향적 코호트 연구에서 일주일 2회 이상 운동을 하는 군과 그 미만으로 운동을 하는 군으로 나누어 뇌구조 자기공명영상을 시행한 결과, 운동을 자주 했던 군에서 전두엽 부위에 회색질(grey matter)의 부피가 적은 운동을 했던 군보다 더 높게 측정된 연구가 있다.22) 신체 활동을 통해 노인에게는 해마(hippocampus) 부피의 증가가 일어날 수 있으며 인지기능 영역 중 기억(memory function)의 향상이 일어날수 있다.23) 메타분석에서는 학령기부터 노인에 이르기까지 유산소 운동으로 조절능력(inhibitory control), 인지적 유연성(cognitive flexibility), 작업기억(working memory)의 영역이 향상될 수 있음이 밝혀졌다.24)
신체활동이 BDNF 유전자의 발현을 증가시키는 기전은 NMDA 수 용체를 통한 CaMKII의 활성 증가, AMP/ATP radtio 상승으로 인한 SIRTI의 활성화로 후성유전학적 조절을 통해 이루어진다.25) 또한 신체활동은 CREB-BDNF 신호전달 과정을 조절하는 microRNA-132 (miR-132), microRNA-134(miR-134)를 조절하여 시냅스의 신경전달 활성화와 BDNF의 증가를 유도할 수 있다.26)27) 흥미롭게도 신체활동을 통한 후성 유전학적 조절은 인간의 정자 발생시 DNA 메틸화(methylation)에 영향을 주어 후대에서 조현병, 파킨슨병과 관련된 유전자의 발현을 억제하고,28) miRNA의 발현의 변화는 설치류 연구에서 정자 발생시 miRNA 발현에 영향을 주어 후대에서 공포반응 및 불안반응을 줄인다는 연구가 있다.29) 따라서 신체활동을 통해 뇌 신경가소성을 높일 수 있으며 이러한 기전으로 정신과적 증상 및 질환, 신경변성질환 을 예방할 수 있다.
간헐적 단식이나 열량 제한(calorie restriction)은 노화와 관련된 인지기능의 저하와, 알츠하이머병, 파킨슨병과 같은 신경변성질환의 발생을 지연시킨다 는 연구가 있다.30) 시냅스의 항상성과 뇌 신경가소성의 증가, 대사성 질환과 산화스트레스(oxidative stress)에 대한 저항을 통해 신경세포의 생성과 기능이 유지된다.31)32) 또한 열량 제한은 치아이랑(dentate gyrus)에서 BDNF의 생성, 신경발생, 신규 신경세포의 생존을 증가시킨다.33) 반대로 고열량, 고지방, 정제당(액상과당, 설탕)의 섭취가 증가하면서 해마의 BDNF 생성이 감소하고 이로 인해 뇌 신경가소성, 신경발생이 저하된다는 연구도 있다.34) 이러한 연관성으로 최근 비만, 식이습관, 당뇨병과 치매가 연관이 있다는 견해가 있으며 이러한 알츠하이머병을 제3형 당뇨(type 3 diabetes)로 제안하기도 한다.35) 하지만 식이 습관이 BDNF의 생성과 뇌 신경가소성에 영향을 미치는 기전은 아직 명확히 밝혀지지 않았다.
Omega-3 지방산, 철분, 아연, vitamin E는 BDNF의 생성에 도움을 주며 이를 통해 뇌 신경가소성을 증가시킬 수 있다. Omega-3 지방산은 부족한 경우 인지기능의 저하와 알츠하이머병, 조현병, 우울증, ADHD의 발생을 증가시키는 것으로 알려져 있다.36)37) Omega-3 지방산은 BDNF-CREB 신호 전달 체계를 통해 낮은 BDNF의 농도를 정상화시킨다. 따라서 별다른 질환이 없는 경우 omega-3 지방산의 효과는 미미할 수 있다.38) Vitamin E는 항산화 효과가 있고 이를 통해 뉴런의 산화 스트레스를 낮추어 신경세포 보호의 효과를 보이며, omega-3 지방산과 같이 BDNF-CREB 신호전달 체계를 통해 낮은 BDNF의 농도를 정상화시켜 알츠하이머병, 우울증, 양극성 장애의 발병을 억제한다.39)40) 철분은 헤모글로빈을 구성하는 미네랄로 산소 운반, 신경전달물질의 합성에 필요하다. 체내 철분의 농도가 높은 경우에도 병리생태학적 문제가 발생하고 신경세포의 변성이 일어날 수 있다. 하지만 농도가 낮은 경우도 해마에서 BDNF 유전자의 발현이 감소하고 이로 인해 신경발생, 뉴런의 기능의 저하를 일으킨다.41) 아연은 체내 효소의 보조인자로 작용하고 특히 metalloprotein의 보조인자로 작용한다. 이는 시냅스 신호전달과 세포 간 신호전달 체계에 중요한 역할을 한다. 아연은 mature BDNF의 형성에 관여하는 MMP-2와 MMP-9의 보 조인자로서 뇌 신경가소성을 향 상시킬 수 있다.42) 아연의 경우 추가적 섭취를 통한 정신과 질환의 예방 및 치료의 효과는 현재까지 알려진 바는 없다. 하지만 조현병 환자에서 Positive and Negative Syndrome Scale (PANSS) 총점과 혈중 아연 농도 간에 음의 상관관계를 보인다는 연구가 있어 향후 아연의 치료적 효과 및 임상적 의미에 대한 추가 연구가 필요하다.43)
사람을 비롯한 포유류는 생활환경이 삶의 질과 질병의 발생에 영향을 미친다. 환경 풍부화의 개념은 동물사육 분야에서 처음 개발된 개념이며 생활 환경의 최적화 를 바탕으로 하고 있다. 적절한 넓이의 생활공간에서 다양한 시각, 청각, 촉각, 협응운동자극을 주고 개체 간 사회적 교류를 수행할 수 있는 정도로 개체수를 조정하여 생활 환경을 최적화한다. 환경 조정을 통해 유전자 발현에서 후성유전학적 변화가 발생하고 이는 뇌의 발달과 정신건강의학과 질환의 발생에 영향을 준다.44) 동물 대상 연구에서 환경 풍부화를 통해 시냅스, 뉴런의 생성이 증가 됨을 확인하였다.45) 또한 정신건강의학과 질환 모델 설치류의 연구에서 환경 풍부화로 우울증, 불안장애의 발생이 감소하고 이는 후성유전학적으로 전달되어 자녀 세대에도 지속되는 것으 로 밝혀졌다.46) 최근 사람을 대상으로 연구가 진행되고 있으며 정상인, 알츠하이머병 및 신경변성질환의 환자군에서 다양한 자극과 인지훈련, 사회적 교류를 증가시킴으 로써 BDNF의 증가가 관찰되었다.47) 환경 풍부화를 사람을 대상으로 적용하는데 거주 공간 최적화의 개념에서 어려움이 있으나 주변의 적절한 자극, 사회적 교류, 운동, 인지 자극과 같은 변화 가능한 환경 요인에 대한 연구가 활발히 진행 중에 있다.
뇌에 직접적인 전기, 자기장을 주어 이를 통해 기능을 회복하고 증상을 치료하는 뉴로모듈레이션은 고전적인 방식의 전기경련치료(electroconvulsive therapy, ECT)에서부터 발전 되었다. 최근에는 의식 소실 없이 뉴런의 기능 및 생리적 변화를 유도하여 우울증, 인지기능장애 등의 질환에 치료적 효과를 보이는 TMS, tDCS가 새로운 치료의 대안으로 연구되고 있다. 임상적으로 적용하며 TMS의 효과를 확인하였으나 명확한 작용기전은 알려지지 않았다. TMS로 발생하는 생물학적 변화를 측정한 연구들에서 가장 일관되게 설명되는 부분은 BDNF의 변화가 발생하 는 것이다.48)49) TMS는 뇌손상으로 발생하 는 기능장애, 우울증, 인지기능장애에 BDNF-TrkB 신호전달체계를 활성화시켜 임상적 효과를 보인다.50) 하지만 TMS는 대상자의 BDNF 유전자에 Val66Met SNP가 있을 경우 치료 효과가 떨어질 수 있으며 시술 후 발생하는 일시적 기억장애의 부작용의 확률이 높아질 수 있다.51 tDCS는 TMS에 비해 상대적으로 사용된 기간이 짧지만 최근 TMS 보다 많은 연구가 이루어지고 있다.
tDCS의 작용 기전은 NMDA와 Ca2+의 세포 내 유입으로 BDNF의 발현에 영향을 주어 뇌 신경가소성에 영향을 주고 신경세포의 활성화를 유도하 는 것으로 알려져 있다.52) tDCS도 우울증의 우울증상, 조현병의 환각을 줄일 수 있다는 연구가 있으나 아직 소규모 연구가 대부분이다. 우울증의 신체적 증상인 수면장애, 체중 변화를 호전시키지 못하는 것으로 알려져 있고 연구자 간 우울증상을 호전시키는 부분에 있어서도 결과가 혼재되어 있다.53) 또한 조현병에서도 환청에 대한 치료 효과가 유의하지 않다는 연구도 있다.54) 향후 추가적인 연구를 통해 tDCS의 임상적 치료효과에 대한 입증이 필요하다.
신경세포의 소실, 변성, 기능적 이상을 뇌 신경가소성을 통해 예방하고 정신과적 증상을 조절할 수 있다. 뇌 신경가소성의 생물학적인 기전은 주로 BDNF 유전자의 발현 조절과 생성된 인자의 작용을 통한 신경세포의 재생, 유지임을 확인 할 수 있다. 이러한 생물학적기전을 활성화하는 비약 물학적 요인에 대한 리뷰를 진행하였다. 운동, 영양 학적 요인 , 환경 풍부화, 뉴로 모듈레이션이 비약 물적으로 BDNF 유전자의 발현을 조절하고 뇌 신경가소성을 향상시킬 수 있다. 결과적으로 임상에서 우울증, 인지기능장애 환자에게 약물적 치료 이외에 운동, 식습관 교정, 환경 풍부화를 적용하도록 권고하고, 필요하다면 뉴로모듈레이션을 권하는 것은 부작용의 측 면에서 안전하고, 약 물치료와 병행하였을 때 시너지를 일으키는 효과적인 치료적 접근이 될 수 있다. 향후 뉴로모듈레이션의 정확한 작용기전, 적절한 시술 프로토콜에 대한 연구가 필요하며, 사람을 대상으로 하는 환경 풍부화에 대한 연구가 진행되어야 할 것이다. 또한 미래에는 Va l6 6Met SN P에 따른 각각의 치료 효과 및 부작용 프로파일에 대한 추가적인 연구가 진행되어 임상현장에서 환자의 유전적인 특성에 따른 맞춤 의학을 적용할 수 있기를 기대한다.
중심 단어: 뇌 신경가소성; 뇌유래신경영양인자; 비약물적 개입.
Acknowledgments
None
Conflicts of interest
The authors have no financial conflicts of interest
Author Contributions
Conceptualization: Nak-Young Kim. Supervision: Hyun Kook Lim. Writing—original draft: Nak-Young Kim. Writing—review & editing: Hyun Kook Lim.
ORCID iDs
Nak-Young Kim https://orcid.org/0000-0003-0116-6283
Hyun Kook Lim https://orcid.org/0000-0001-8742-3409
1) Wang CS, Kavalali ET, Monteggia LM. BDNF signaling in context: from synaptic regulation to psychiatric disorders. Cell 2022;185:62-76.
2) Burke SN, Barnes CA. Neural plasticity in the ageing brain. Nat Rev Neurosci 2006;7:30-40.
3) Azman KF, Zakaria R. Recent advances on the role of brain-derived neurotrophic factor (BDNF) in neurodegenerative diseases. Int J Mol Sci 2022;23:6827.
4) Abdanipour A, Moradi F, Fakheri F, Ghorbanlou M, Nejatbakhsh R. The effect of lithium chloride on BDNF, NT3, and their receptor mRNA levels in the spinal ontusion rat models. Neurol Res 2019; 41:577-583.
5) Mosiołek A, Mosiołek J, Jakima S, Pięta A, Szulc A. Effects of anti-depressant treatment on neurotrophic factors (BDNF and IGF-1) in patients with major depressive disorder (MDD). J Clin Med 2021; 10:3377.
6) Kang MJY, Hawken E, Vazquez GH. The mechanisms behind rapid antidepressant effects of ketamine: a systematic review with a focus on molecular neuroplasticity. Front Psychiatry 2022;13:860882.
7) Sarris J, O’Neil A, Coulson CE, Schweitzer I, Berk M. Lifestyle medicine for depression. BMC Psychiatry 2014;14:107.
8) Rostamzadeh A, Kahlert A, Kalthegener F, Jessen F. Psychotherapeutic interventions in individuals at risk for Alzheimer’s dementia: a systematic review. Alzheimers Res Ther 2022;14:18.
9) Zhao C, Deng W, Gage FH. Mechanisms and functional implications of adult neurogenesis. Cell 2008;132:645-660.
10) Branchi I, Francia N, Alleva E. Epigenetic control of neurobehavioural plasticity: the role of neurotrophins. Behav Pharmacol 2004; 15:353-362.
11) Waterhouse EG, An JJ, Orefice LL, Baydyuk M, Liao GY, Zheng K, et al. BDNF promotes differentiation and maturation of adult-born neurons through GABAergic transmission. J Neurosci 2012;32:14318-14330.
12) Carter AR, Chen C, Schwartz PM, Segal RA. Brain-derived neurotrophic factor modulates cerebellar plasticity and synaptic ultrastructure. J Neurosci 2002;22:1316-1327.
13) Balaratnasingam S, Janca A. Brain derived neurotrophic factor: a novel neurotrophin involved in psychiatric and neurological disorders. Pharmacol Ther 2012;134:116-124.
14) Deinhardt K, Chao MV. Shaping neurons: long and short range effects of mature and proBDNF signalling upon neuronal structure. Neuropharmacology 2014;76(Pt C):603-609.
15) Patel AV, Krimm RF. BDNF is required for the survival of differentiated geniculate ganglion neurons. Dev Biol 2010;340:419-429.
16) Chen SD, Wu CL, Hwang WC, Yang DI. More insight into BDNF against neurodegeneration: anti-apoptosis, anti-oxidation, and suppression of autophagy. Int J Mol Sci 2017;18:545.
17) Yang JL, Lin YT, Chuang PC, Bohr VA, Mattson MP. BDNF and exercise enhance neuronal DNA repair by stimulating CREB-mediated production of apurinic/apyrimidinic endonuclease 1. Neuro-molecualr Me d 2014;16:161-174.
18) Martens L, Herrmann L, Colic L, Li M, Richter A, Behnisch G, et al. Met carriers of the BDNF Val66Met polymorphism show reduced Glx/NAA in the pregenual ACC in two independent cohorts. Sci Rep 2021;11:6742.
19) Szarowicz CA, Steece-Collier K, Caulfield ME. New frontiers in neurodegeneration and regeneration associated with brain-derived neurotrophic factor and the rs6265 single nucleotide polymorphism. Int J Mol Sci 2022;23:8011.
20) Pathak P, Mehra A, Ram S, Pal A, Grover S. Association of serum BDNF level and Val66Met polymorphism with response to treatment in patients of major depressive disease: a step towards personalized therapy. Behav Brain Res 2022;430:113931.
21) Cheng CM, Hong CJ, Lin HC, Chu PJ, Chen MH, Tu PC, et al. Predictive roles of brain-derived neurotrophic factor Val66Met polymorphism on antidepressant efficacy of different forms of prefrontal brain stimulation monotherapy: a randomized, double-blind, sham-controlled study. J Affect Disord 2022;297:353-359.
22) Rovio S, Kåreholt I, Helkala EL, Viitanen M, Winblad B, Tuomilehto J, et al. Leisure-time physical activity at midlife and the risk of dementia and Alzheimer’s disease. Lancet Neurol 2005;4:705-711.
23) Mahalakshmi B, Maurya N, Lee SD, Bharath Kumar V. Possible neuroprotective mechanisms of physical exercise in neurodegeneration. Int J Mol Sci 2020;21:5895.
24) Ludyga S, Gerber M, Brand S, Holsboer-Trachsler E, Pühse U. Acute effects of moderate aerobic exercise on specific aspects of executive function in different age and fitness groups: a meta-analysis. Psycho phy siolog y 2016;53:1611-1626.
25) Fernandes J, Arida RM, Gomez-Pinilla F. Physical exercise as an epigenetic modulator of brain plasticity and cognition. Neurosci Biobehav Rev 2017;80:443-456.
26) Wayman GA, Davare M, Ando H, Fortin D, Varlamova O, Cheng HY, et al. An activity-regulated microRNA controls dendritic plasticity by down-regulating 250GAP. Proc Natl Acad Sci U S A 2008; 105:9093-9098.
27) Gao J, Wang WY, Mao YW, Gräff J, Guan JS, Pan L, et al. A novel pathway regulates memory and plasticity via SIRT1 and miR-134. Nat u re 2010;466:1105-1109.
28) Denham J, O’Brien BJ, Harvey JT, Charchar FJ. Genome-wide sperm DNA methylation changes after 3 months of exercise training in humans. Epigenomics 015;7:717-731.
29) Short AK, Yeshurun S, Powell R, Perreau VM, Fox A, Kim JH, et al. Exercise alters mouse sperm small noncoding RNAs and induces a transgenerational modification of male offspring conditioned fear and anxiety. Transl Psychiatry 2017;7:e1114.
30) Seidler K, Barrow M. Intermittent fasting and cognitive performance – Targeting BDNF as potential strategy to optimise brain health. Front Neuroendocrinol 2022;65:100971.
31) Guo Z, Ersoz A, Butterfield DA, Mattson MP. Beneficial effects of dietary restriction on cerebral cortical synaptic terminals: preservation of glucose and glutamate transport and mitochondrial function after exposure to amyloid beta-peptide, iron, and 3-nitropropionic acid. J Neurochem 2000;75:314-320.
32) Gomez-Pinilla F, Tyagi E. Diet and cognition: interplay between cell metabolism and neuronal plasticity. Curr Opin Clin Nutr Metab Care 2013;16:726-733.
33) Lee J, Duan W, Long JM, Ingram DK, Mattson MP. Dietary restriction increases the number of newly generated neural cells, and induces BDNF expression, in the dentate gyrus of rats. J Mol Neurosci 200 0;15:99-108.
34) Molteni R, Barnard RJ, Ying Z, Roberts CK, Gómez-Pinilla F. A high-fat, refined sugar diet reduces hippocampal brain-derived neurotrophic factor, neuronal plasticity, and learning. Neuroscience 2002;112:803-814.
35) Selman A, Burns S, Reddy AP, Culberson J, Reddy PH. The role of obesity and diabetes in dementia. Int J Mol Sci 2022;23:9267.
36) Conquer JA, Tierney MC, Zecevic J, Bettger WJ, Fisher RH. Fatty acid analysis of blood plasma of patients with Alzheimer’s disease, other types of dementia, and cognitive impairment. Lipids 2000;35:
1305-1312.
37) Ortega RM, Rodríguez-Rodríguez E, López-Sobaler AM. Effects of omega 3 fatty acids supplementation in behavior and non-neuro-degenerative neuropsychiatric disorders. Br J Nutr 2012;107(Suppl 2):S261-S270.
38) Pieramico V, Esposito R, Cesinaro S, Frazzini V, Sensi SL. Effects of non-pharmacological or pharmacological interventions on cognition and brain plasticity of aging individuals. Front Syst Neurosci
2014;8:153.
39) Cecerska-Heryć E, Polikowska A, Serwin N, Roszak M, Grygorce-wicz B, Heryć R, et al. Importance of oxidative stress in the pathogenesis, diagnosis, and monitoring of patients with neuropsychiatric disorders, a review. Neurochem Int 2022;153:105269.
40) Wu A, Ying Z, Gomez-Pinilla F. The interplay between oxidative stress and brain-derived neurotrophic factor modulates the outcome of a saturated fat diet on synaptic plasticity and cognition. Eur J
Neurosci 2004;19:1699-1707.
41) Mehrpouya S, Nahavandi A, Khojasteh F, Soleimani M, Ahmadi M, Barati M. Iron administration prevents BDNF decrease and depressive-like behavior following chronic stress. Brain Res 2015;1596:79-87.
42) Taked a A. Movement of zinc and its functional significance in the brain. Brain Res Brain Res Rev 2000;34:137-148.
43) Zaks N, Austin C, Arora M, Reichenberg A. Reprint of: elemental dysregulation in psychotic spectrum disorders: a review and research synthesis. Schizophr Res 2022;247:33-40.
44) Rule L, Yang J, Watkin H, Hall J, Brydges NM. Environmental enrichment rescues survival and function of adult-born neurons following early life stress. Mol Psychiatry 2021;26:1898-1908.
45) Ohline SM, Abraham WC. Environmental enrichment effects on synaptic and cellular physiology of hippocampal neurons. Neuro-pharmacology 2019;145(Pt A):3-12.
46) Sparling JE, Barbeau K, Boileau K, Konkle ATM. Environmental enrichment and its influence on rodent offspring and maternal behaviours, a scoping style review of indices of depression and anxiety. Pharmacol Biochem Behav 2020;197:172997.
47) Cutuli D, Landolfo E, Petrosini L, Gelfo F. Environmental enrichment effects on the brain-derived neurotrophic factor expression in healthy condition, Alzheimer’s disease, and other neurodegenerative disorders. J Alzheimers Dis 2022;85:975-992.
48) Tong J, Zhang J, Jin Y, Liu W, Wang H, Huang Y, et al. Impact of repetitive transcranial magnetic stimulation (rTMS) on theory of mind and executive function in major depressive disorder and its correlation with brain-derived neurotrophic factor (BDNF): a randomized, double-blind, sham-controlled trial. Brain Sci 2021;11:765.
49) McNerney MW, Heath A, Narayanan SK, Yesavage J. Repetitive transcranial magnetic stimulation improves brain-derived neuro-trophic factor and cholinergic signaling in the 3xTgAD mouse model of Alzheimer’s disease. J Alzheimers Dis 2022;86:499-507.
50) Wang HY, Crupi D, Liu J, Stucky A, Cruciata G, Di Rocco A, et al. Repetitive transcranial magnetic stimulation enhances BDNF-TrkB signaling in both brain and lymphocyte. J Neurosci 2011;31:11044-
110 54.
51) Abellaneda-Pérez K, Martin-Trias P, Cassé-Perrot C, Vaqué-Alcázar L, Lanteaume L, Solana E, et al. BDNF Val66Met gene poly-morphism modulates brain activity following rTMS-induced memory impairment. Sci Rep 2022;12:176.
52) Podda MV, Cocco S, Mastrodonato A, Fusco S, Leone L, Barbati SA, et al. Anodal transcranial direct current stimulation boosts synaptic plasticity and memory in mice via epigenetic regulation of Bdnf expression. Sci Rep 2016;6:22180.
53) Chase HW, Boudewyn MA, Carter CS, Phillips ML. Transcranial direct current stimulation: a roadmap for research, from mechanism of action to clinical implementation. Mol Psychiatry 2020;25:39 7-407.
54) Fitzgerald PB, McQueen S, Daskalakis ZJ, Hoy KE. A negative pilot study of daily bimodal transcranial direct current stimulation in schizophrenia. Brain Stimul 2014;7:813-816.